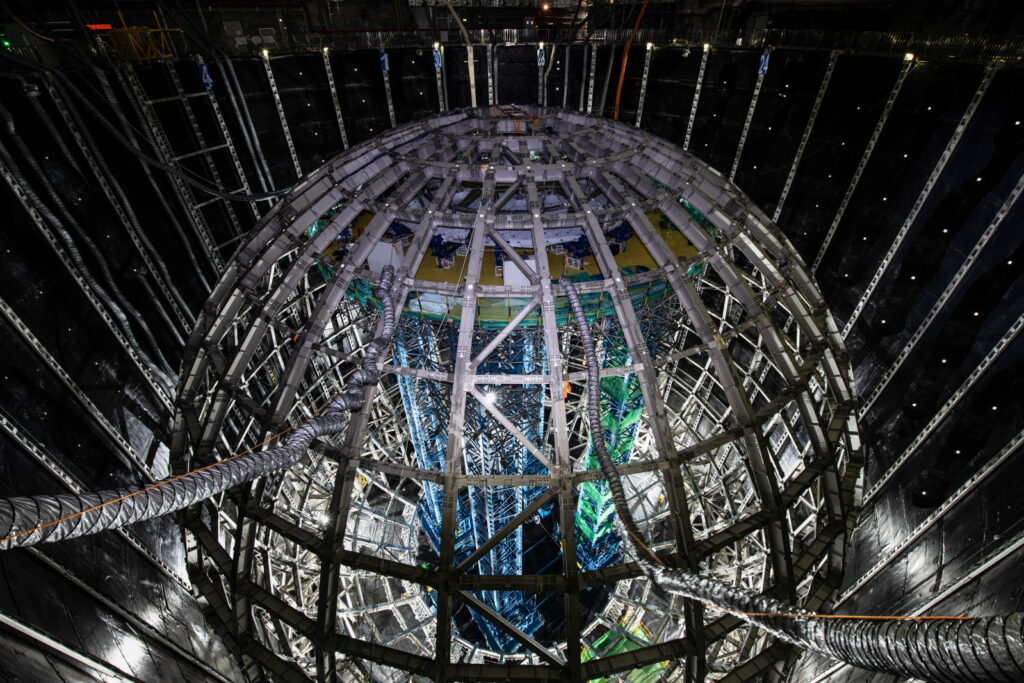
The Jiangmen Underground Neutrino Observatory (JUNO) is a large liquid scintillator neutrino experiment whose main goal is to act as a multipurpose observatory for neutrinos produced by artificial and natural sources. JUNO is currently in construction in the Jiangmen underground facility, near the city of Kaiping, in Southern China. This location is really peculiar since it is at about 53 km from both the nuclear power plants of Yangjiang (6 reactors, 2.9 GW$_{\mathrm{th}}$ each) and Taishan (2 reactors, 4.6 GW$_{\mathrm{th}}$ each), and since there is no other nuclear power plant within a 200 km radius. Due to the very small neutrino interaction rate, the shielding against cosmic rays and the experiment extreme radiopurity are mandatory. To suppress the muon induced backgrounds, the JUNO detector is located below an average rock overburden of about 650 m, resulting in a shielding capacity against cosmic rays of about 1800 m.w.e.
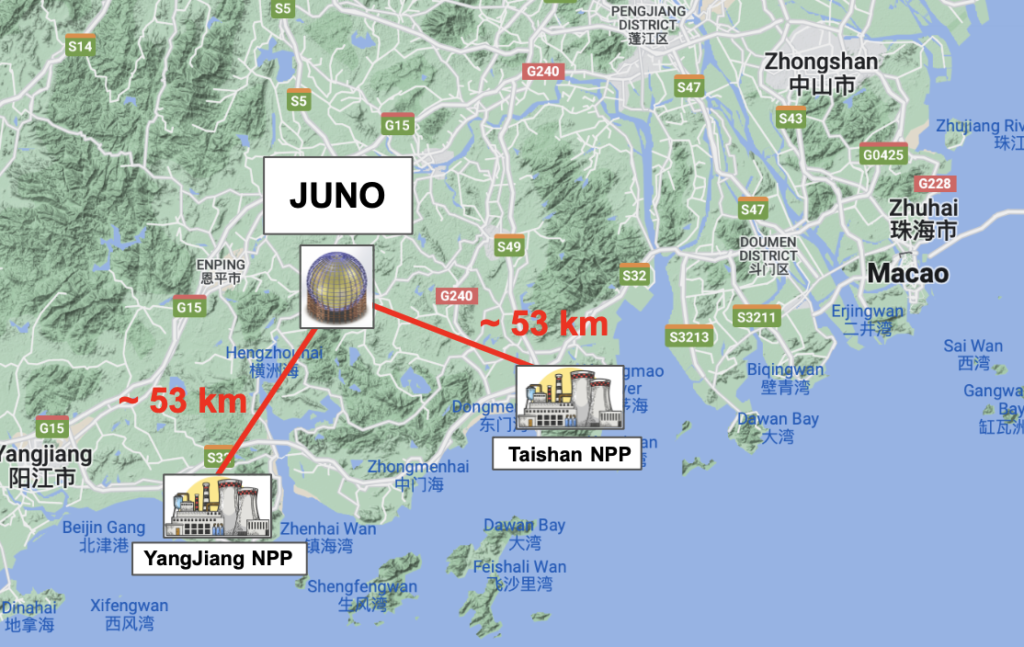
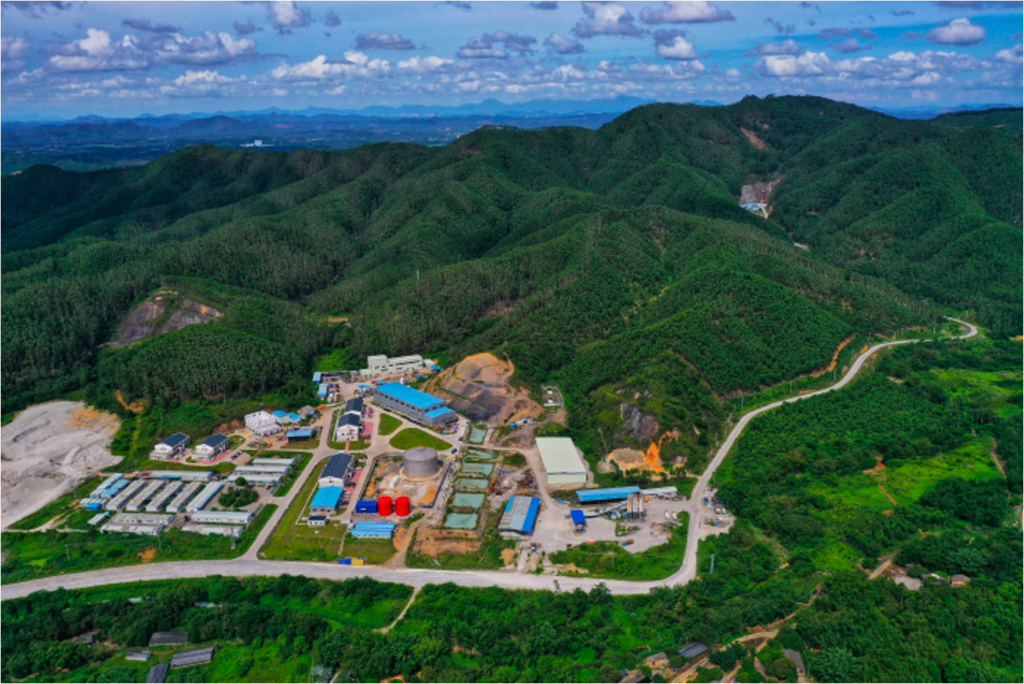
The primary JUNO scientific goal is the determination of the neutrino mass ordering (NMO). This can be inferred by measuring the oscillation pattern of electron anti-neutrinos emitted by nuclear power plants. To reach this ambitious goal, JUNO will benefit of a large 20 kton scintillator detection mass, and will target an unprecedented 3% energy resolution at 1 MeV scale. Sensitivity studies show that a $3\sigma$-$4\sigma$ statistical significance on NMO can be reached in six years of data-taking.

Thanks to its unique features, the JUNO scientific program extends to several astroparticles physics goals such as the solar neutrino precision spectroscopy, the sub-percent determination of flavour oscillation parameters sin$2\theta_{12}$, $\Delta \mathrm{m}^2_{12}$ and $\Delta \mathrm{m}^2_{13}$, the measurements of atmospheric neutrinos, geo-neutrinos, supernovae neutrinos, Non Standard Interaction neutrinos and other exotic searches.
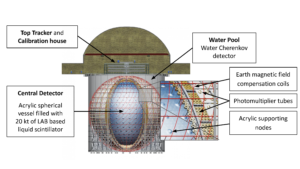
In order to fulfil its very rich physics program, JUNO has to target the highest radiopurity from its very beginning. The detector structure, as shown above, will exploit the principle of graded-shielding: the more moving towards the center of the detector, the radiopurer the detector will be. Starting from the external, there is the Top Tracker with the calibration house and the Water Pool, which will act as gamma and neutron shield as well as water-Cherenkov detector. Then there is the Central detector Support Structure on which the photomultipliers are installed in a specific geometric pattern that will guarantee the maximum geometric coverage. Finally, the acrylic spherical vessel which will contain the core of the JUNO experiment: 20-kt of organic linear alkyl benzene (LAB) based liquid scintillator.
The top tracker’s goals are to provide a precise muon tracking and to study the cosmogenic background. The JUNO top tracker will cover about 60% of the water pool top surface and, thus, will be able to monitor about one-third of all atmospheric muons passing through the central detector. The detector medium of the top tracker will consist of three layers of plastic scintillator panels (dimensions: $\approx~40\mathrm{\,m}\times\,20\mathrm{\,m}\times\,3\mathrm{\,m}$), which were inherited from the OPERA experiment target tracker.
JUNO will profit from an advanced calibration system to test and study the energy scale, the detector response non-uniformity and the energy non-linearity. These crucial items will be achieved thanks to three different scan systems: the 1D automatic calibration unit, the 2D cable loop system and guide tube calibration system, and the 3D remotely operated vehicle. Several radioactive sources ($\gamma$, e$^+$, n) with energy covering from 0.5 to about 8-10 MeV will be used in order to fully map the JUNO energy region of interest.
The JUNO water pool will contain the central detector, and about 35 kt of ultra pure water, aiming to protect the central detector itself from natural radioactivity in surrounding rocks. Being equipped with 2400 20″-PMTs, the water pool will also serve as water Cherenkov detector to tag cosmic muons Cherenkov light, with an efficiency of more than 99.5% [JUNO coll. 2016]. The water pool was constructed according to strict requirements of low radon radioactivity, stable temperature so to prevent any convective motion of the water, and an attenuation length of 35 m.
Inside the water pool there is a steel support structure (around 600~t) of about 40 m diameter which will support the PMTs and bear the JUNO acrylic vessel. The acrylic sphere encloses the scintillator. It is a 35.4 m diameter sphere, with a weight of about 600~t, designed and selected for its high radiopurity and optimal optical features.
The JUNO experiment will use a huge number of photomultipliers: besides the 2400 20″-PMTs in the water pool, 17612 20″-PMTs and 25600 3″-PMTs will be installed in the central detector as per the scheme depicted below.
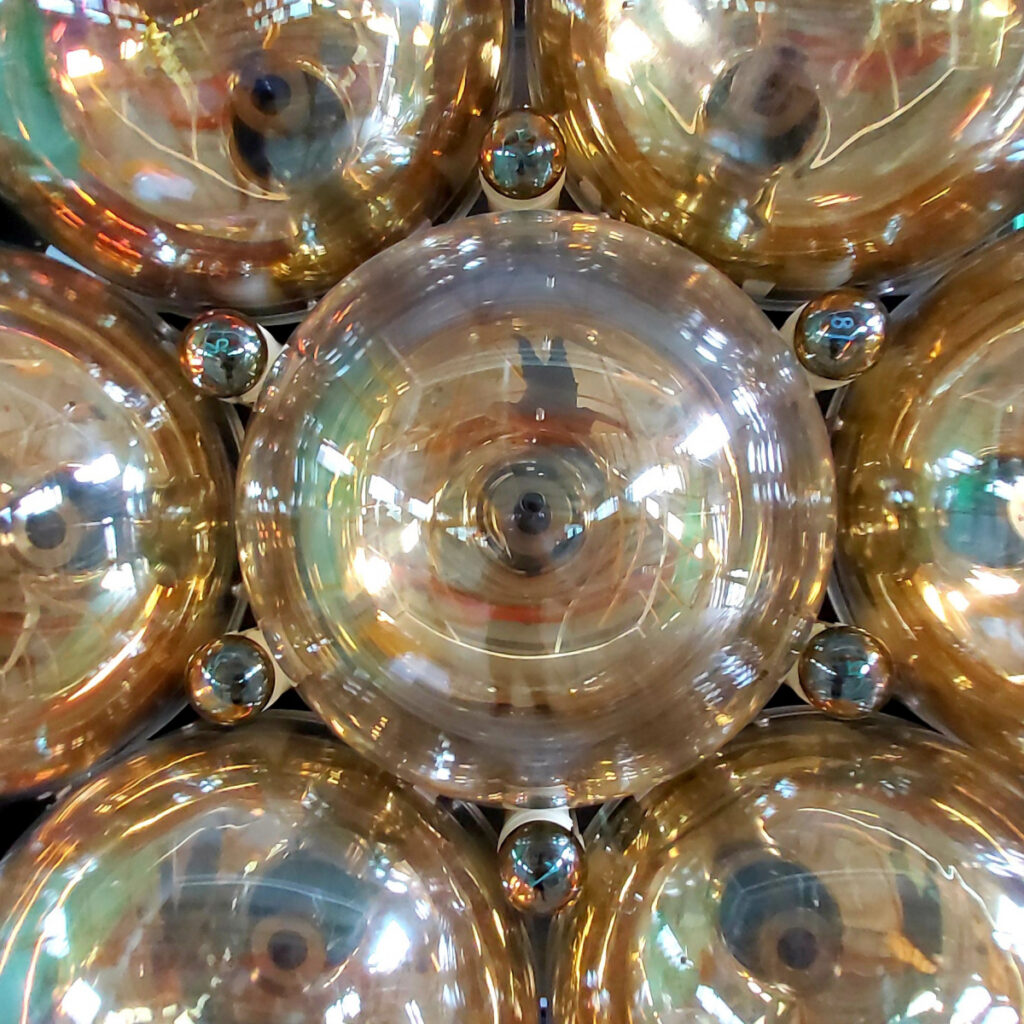
Their photon detection efficiency was evaluated to be $\sim$30% and $\sim$25% for the 20″ and the 3″ PMTs, respectively. The PMTs assembly precision will be very tight (< 1mm) and the final clearance between photomultipliers will be an impressive 3mm: this will guarantee JUNO a PMT geometric coverage of about 78% (75.2% and 2.7%, for the 20″ and the 3″ PMTs, respectively), the largest ever achieved so far. JUNO will profit of an optimal light level for a PMT-based detector: indeed, the expected light-yield value is 1345 p.e./MeV [JUNO coll. 2021]. Moreover, recent studies have shown that, thanks to refinements to the optical model, and improvements on the central detector geometries, the JUNO light-yield could further increase to an unprecedented 1665 p.e./MeV. All PMTs have already been produced, tested and instrumented with waterproof potting. The related electronics is specially custom-made and it is installed close to the PMTS, i.e. underwater, to improve and maximize the signal-to-noise ratio.
The JUNO liquid scintillator is an organic mixture of linear alkyl benzene as solvent, plus a fluor (PPO, 2.5 g/L), and a wavelength shifter (bis-MSB, 3 mg/L) as solutes. The strict background constraints to perform reactor and solar physics ($10^{-15}-10^{-17}$ g/g in $^{238}$U and $^{232}$Th, respectively) call for the JUNO scintillator to be extremely radiopure. Moreover, the scintillator will have to show a high light yield ($\sim10^4\;$photon/MeV) and an attenuation length of more than 20 m at the characteristic light emission ($\mathrm{\lambda_{avg}=430~nn}$). Prior to its insertion into the JUNO acrylic sphere, the 20-kt liquid scintillator will be purified onsite with Alumina (Al$_2$O$_3$) filtration to remove particulates, distillation and water extraction to remove radioactive impurities, and gas stripping to remove Radon and Oxigen. Before and during the filling, part of the scintillator (about 15%) will be analyzed in a dedicated facility named OSIRIS in order to test and verify the radiopurity constraints.